Liheng Cai
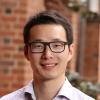
About
My lab’s research lies at the interface of soft (bio)materials and biology. We seek to understand and control the interactions between soft (bio)materials and living systems to solve challenges in sustainability and health. We achieve this through a combination of experimental and theoretical approaches. Our core expertise includes polymers and soft matter, biomaterials, voxelated bioprinting, and additive manufacturing of soft and inorganic matter. Our research program is highly collaborative and interdisciplinary. The philosophy of our research is to identify and solve problems of both fundamental importance and practical value; this is often accomplished by working closely with experts from various fields.
Currently, we are pursuing research in three fields: (1) polymers and soft matter, (2) advanced (bio)manufacturing, and (3) bioengineering, each of which integrates synthesis, theory, experiment, and translation. In addition to practical applications in sustainability and biomedicine, we hope to answer three grand scientific questions:
- What are the molecular mechanisms for nonlinear (mechanical, electric, magnetic, and optical) properties of soft materials under large deformations?
- How do soft (bio)materials interact with biological objects?
- Can we use polymer physics and soft matter principles to understand the rules of life?
Education
B.S. Physics, Lanzhou University, 2006
Ph.D. Materials Science, University of North Carolina at Chapel Hill, 2012
Postdoctoral Fellow, Harvard University, 2013-2017
"We aim to understand and control the interactions between soft (bio)materials and living systems to solve challenges in sustainability and health."