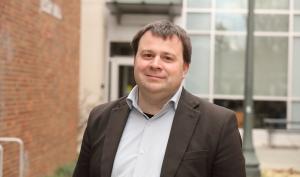
Catalysts are tricky things, but without them, our world would be a lot dirtier.
Catalysts – materials that accelerate chemical reactions – are used to save energy, reduce waste and control pollution in everything from manufacturing household cleaners to making car emissions less toxic.
It’s a tough life, though. These materials operate in hostile environments that can degrade their ability to work efficiently, or stop them from functioning at all. That’s what makes them tricky.
“A primary bottleneck in many applications has been stability of the catalyst,” said Chris Paolucci, an assistant professor of chemical engineering at the University of Virginia School of Engineering and Applied Science. “Let’s say I synthesize something perfectly. I make the material the exact way I want it, but when I start running my chemical reaction that I’m interested in, everything changes. I end up getting something I didn’t start with, and I don’t really understand why that is.”
Paolucci recently received a National Science Foundation CAREER Award to help figure out what happens to catalytic materials during reaction, down to their nanoparticles, atoms and ions. This understanding is the key to designing longer-lasting, efficient catalysts that work in a variety of conditions.
The CAREER program, one of the NSF’s most prestigious awards for early-career faculty, recognizes the recipient’s potential for leadership in research and education. A portion of Paolucci’s five-year, $597,273 grant will cover the cost of computing time on Rivanna, UVA’s high-performance computing system.
Paolucci’s Lab specializes in computer simulations of chemical reactions, including computational catalysis, an area of study that applies quantum mechanics-based calculations to examine how a known catalyst accelerates chemical reactions at the atomic and molecular level, and to build models to predict the performance of potential new catalysts.
Paolucci’s CAREER Award project, “Cation and Nanoparticle Interconversion in Metal-Exchanged Zeolites,” focuses on a widely used class of catalysts that can be designed using any of more than 250 varieties of synthesized zeolites, honeycomb-like mineral frameworks with nano-sized pores. The zeolite structures support metal nanoparticles or positively charged atoms or molecules called cations. The metals provide the “active sites” where reactions occur.
These materials are good at promoting desired reactions, while efficiently using small amounts of expensive metals such as platinum or palladium. Paolucci’s team, led by Ph.D. student Keka Mandal, is looking specifically at catalysts made with those two metals in nanoparticle and cation form.
One complication is that, through a process called interconversion, the conditions in which a catalyst is being used can lead cations to agglomerate, forming metal particles, or, conversely, cause particles to separate into cations. When that happens, the catalyst “deactivates.” The researchers seek to know why, and how to reverse the process to reactivate the material.
To get there, Paolucci’s team will combine quantum mechanical theory – the area of physics dealing with nature’s smallest units of matter – chemical engineering knowhow and computing power. Mandal has logged millions of computing hours on UVA’s supercomputer.
“We work with experimental collaborators on this project, but these interconversions may take 48 hours or longer,” Paolucci said. “To synthesize the zeolite structure and then run an experiment to characterize it can take a long time.”
Instead, his team determines the thermodynamic and kinetic properties of the material using quantum chemical molecular simulations and mathematical modeling.
“That gives us all sorts of different properties for a given molecular system, like energies and forces. Then we use those parameters to build macroscopic models.”
For instance, how much energy does a metal cation have at a given temperature or under a certain pressure – meaning how strongly would that cation bind to a surface under those conditions?
“We work with tools developed by the physics community, but we marry those with core chemical engineering concepts to create computer models that describe the intrinsic thermodynamics and kinetics of a given system. Is the material stable, and if not, how fast do we think it would deactivate or how fast can we make it stable again?”
To help validate and refine the models, Paolucci is collaborating with UVA professor and chair of chemical engineering William Epling’s Environmental Catalysis Lab and professor Rajamani Gounder’s lab at Purdue University. He knows Gounder from his student days at Notre Dame, where Paolucci completed his bachelor’s and Ph.D. degrees in chemical engineering.
Gounder was part of an active catalysis research community that locked in Paolucci’s interest in computational catalysis, first sparked by a computational chemistry class taught by his eventual Ph.D. advisor, Notre Dame professor William Schneider.
“To use computation to predict things like reactions and activation energies, I mean we learned about them in the undergrad curriculum, but to actually be able to visualize those things and compute them, I found really fascinating,” said Paolucci, who brought that enthusiasm to UVA, and not just through research. He also developed and taught a new data science in chemical engineering course.
“But models are an abstraction of reality,” he said. “You’re trying to get as close as you can to the real system, and you can get a lot closer when people are doing fundamental research from the experimental side. I saw a lot of that going on in our field and really appreciated that.”
Together, the experimentalists and modelers can home in on the most important physical parameters, Paolucci said. “At the end of the day, I’m a chemical engineer. Our research is driven by important engineering applications.”