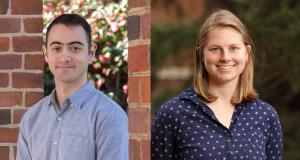
Patrick E. Hopkins, Whitney Stone Professor of Engineering and director of the ExSiTE Lab (Experiments and Simulations in Thermal Engineering), is no stranger to excitement, whether his team is developing a purple freeze ray to create on-demand surface cooling for electronics inside spacecraft and high-altitude jets or heating homes with squid proteins.
But ExSiTE Lab researchers may have outdone themselves.
Hopkins, along with mechanical and aerospace engineering Ph.D. students Milena Milich and Hunter Schonfeld, recently developed a way to recreate the temperature approaching that of a star, approximately 4,000 degrees Kelvin or about 6,740 degrees Fahrenheit, to measure the heat transfer in these extreme conditions.
If you step outside tonight and look at spring's Northern Hemisphere night sky, you can trace the Big Dipper's handle to Arcturus, a red giant dwarf star matching the scorching conditions at ExSiTE lab.
With this amount of heating power, the team was able to measure the thermal conductivity of tungsten at its melting temperature. This is one of the highest temperature thermal conductivity measurements ever reported.
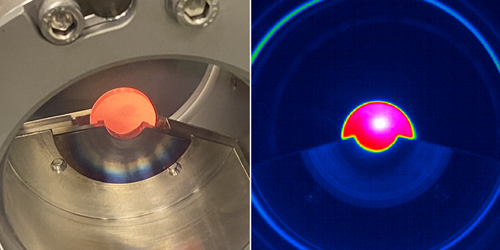
What tungsten looks like when it's melting. (Contributed photo)
The new knowledge of the exact changes in temperature properties at such extremes opens avenues for the creation of materials designed to withstand intense heat fluxes, such as those essential for hypersonic aircraft. It will also help to develop innovative manufacturing techniques to create such craft.
Hopkins will use his U.S. Department of Defense DURIP Award to add sensors and even more higher-powered lasers to the existing thermal conductivity, emissivity (its effectiveness in emitting energy as thermal radiation) and melting temperature capabilities. This equipment will be part of a new laser heating facility at UVA — the only one in the world with the capacity to simultaneously measure this tandem of thermophysical properties at these temperatures.
Their work was published in Physical Review Letters and also featured by APS Physics Magazine, republished with permission below.
Thermal Conductivity Not Too Hot to Handle
by Rachel Berkowitz
April 5, 2024 • Physics 17, s25
A radiometry technique directly measures thermal conductivity in molten metals and confirms the relationship with electrical resistivity.
It’s hard to measure the thermal conductivity of metals at ultrahigh temperatures and in their molten states. It’s so hard, in fact, that rather than directly measuring this property in high-temperature solids, scientists tend to rely on the empirical Wiedemann-Franz law from 1853, which predicts thermal conductivity from electrical resistivity measurements. Milena Milich and Hunter Schonfeld at the University of Virginia and their colleagues have now made the first direct thermal conductivity measurement for nature’s highest-melting-temperature metal: tungsten [1]. Their novel technique not only offers a tool for examining the thermodynamic properties of metals at ultrahigh temperatures without relying on electrical proxies but also provides a means to validate the Wiedemann-Franz law for molten materials.
The researchers used a gradually intensifying laser beam to heat the center of a 2-mm-thick tungsten disk until it began to melt. While they heated the disk, they measured the emitted radiation to determine the temperature of the hot spot. As this region warmed from 2000 to 4000 K (just above tungsten’s melting point), they tracked the incremental temperature change that resulted from each small increase in the laser’s power. From these measurements, a simple calculation provided the thermal conductivity for tungsten at different temperatures. The values matched those in the literature derived from electrical resistivity.
Simulations showed that thermal conductivity is dominated by contributions from electronic transport rather than from phonon vibrations—confirming the accuracy of electrical resistivity as a predictor. This confirmation means that researchers can have confidence in using easier-to-obtain resistivity measurements at the ultrahigh temperatures required for applications in, for example, nuclear fusion and additive manufacturing.